Doping at the Science Museum: the conservation challenge of doped fabric aircraft in the Flight gallery
Abstract
https://dx.doi.org/10.15180/160605/001This article concerns the conservation of historic doped fabric aeroplanes in the static museum setting of the Science Museum’s Flight gallery. It reviews historic sources, primarily archival and scientific research papers, to examine what doped fabric aeroplanes are made from, and why these materials were selected.
It also discusses current conservation methods used for treating tears in the doped fabric covering of aeroplanes, and considers the ethical and practical limitations of these practices. An overview of the doped fabric aircraft collection currently held by the Science Museum is also presented.
Keywords
doped aircraft, doped fabric, Flight gallery, historic aircraft conservation
Introduction
https://dx.doi.org/10.15180/The Science Museum, London, holds an important collection of doped fabric aeroplanes, many of which represent significant technological achievements or events in the history of aviation. Doped fabric aeroplanes were most common in the first half of the twentieth century, and consisted of an internal framework, usually wood or metal, enclosed within a fabric covering. There are several methods by which the fabric could be secured into position, such as using tacks and pins, and sewing the material onto the internal frame (FAA, 1998). This fabric covering would finally be impregnated with a chemical compound, known as ‘dope’, the primary purpose of which was to cause shrinkage of the fabric, thus making it taut and improving the flow of air over it during flight ( Pröll, 1921).
Tears are occurring in this doped fabric covering on some of the aeroplanes on display in the Flight gallery at the Science Museum’s South Kensington site.
The exact causes of these tears are not always known. Some can be directly attributed to human action, principally touching of the material by visitors, while others may be due to repeated humidity fluctuations resulting in cyclical contraction and expansion of the doped fabric. The tearing mechanism may be further complicated by continuing chemical changes in the doped fabric as it ages, which could result in weakening of the material and make tear formation more likely.
This deterioration presents a challenge to staff at the Museum for several reasons. The first is a purely practical issue of gaining access to the tears. Many of the aircraft are displayed hanging from the ceiling and cannot be moved, so reaching areas of damage to assess it and make sufficient room to work can be difficult.
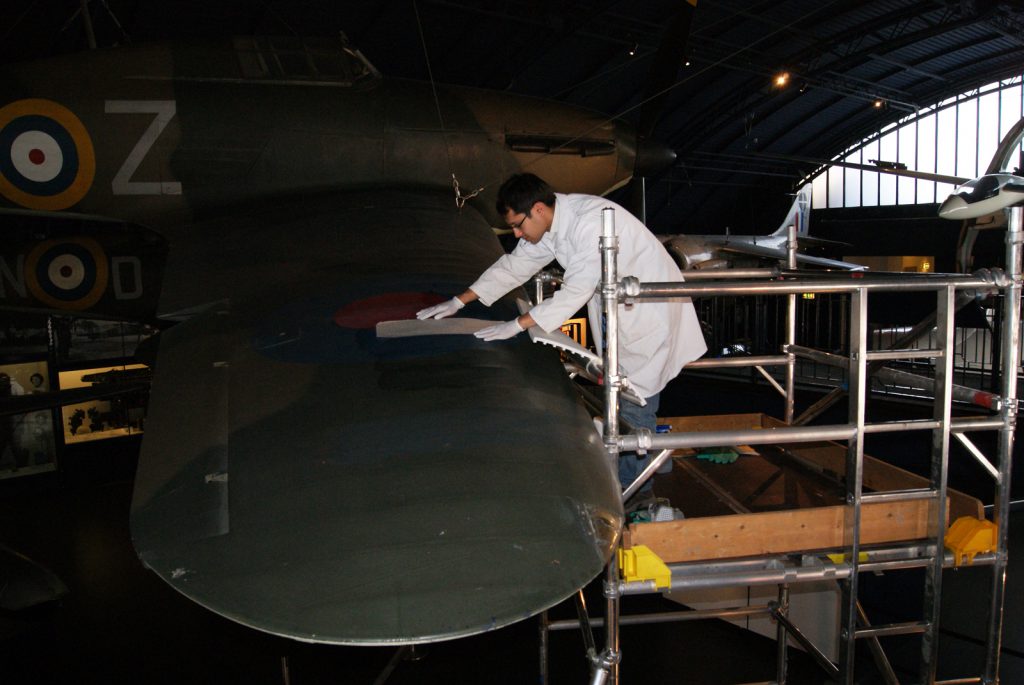
Additionally, the chemical and physical properties of the material are not well understood. This raises uncertainty as to how conservation materials and techniques will react with the doped fabric over time, and there is a risk that well-intentioned treatments may ultimately result in further – potentially more severe – damage.
A doctoral research project jointly conducted by the Science Museum and Imperial College London is therefore under way in an attempt to address some of these issues and identify potential conservation approaches for managing tears in the doped fabric. The project combines a review of archival and historical literature sources with contemporary scientific investigation in order to understand the properties of the doped fabric aeroplanes in the Science Museum’s collection as fully as possible. This improved understanding of the doped fabric will enable more informed choices to be made when deciding on conservation approaches.
The present paper puts forward the findings of a historical literature and archival review to date. Primary archives consulted are those at the Science Museum and the National Archives (NA) at Kew. The Museum archives maintain technical files that relate to the specific objects within the Museum collection, and document all manner of information relating to their history, collection, technology and design. The NA contains documents from the Air Ministry that provide a more general overview regarding the development and use of doped fabric. Much of the historical literature consulted is in the form of scientific investigations and reports into developing doped fabrics and testing their physical properties; other sources, including patents and trade literature, have also proved of value.
The present article begins by providing an overview of what doped fabric is made from and how it was constructed. This is important from a conservation perspective as it may have implications for understanding how and why deterioration takes place. It is also vital for conservation, since without this knowledge making informed choices regarding the conservation materials to use and the methods of repair is much more difficult. This section also provides brief consideration of the types of deterioration that may be associated with these materials, based on both historical observations on the performance of doped fabric and current conservation research into closely related materials.
The article then addresses several of the most common conservation options available for treating tears within the doped fabric skins of aircraft, and the techniques currently used at the Science Museum. This discussion involves some consideration of conservation ethics and the implications different treatment options may entail.
The article finishes by addressing the history and particulars of the doped fabric aircraft within the Museum itself. This is important for a conservation discussion, since the history and previous treatments of objects have implications for how the objects are interpreted and the resulting treatment decisions.
Doped fabric materials
https://dx.doi.org/10.15180/160605/011Understanding how and why particular materials and techniques were chosen for making doped fabric is very important from a conservation perspective for two reasons. The first is due to material identification. In order to manage an object effectively it is crucial to know, or at least be able to make a well-informed assumption, about the types of materials present. This is because identifying the material type may determine conservation treatment decisions, such as the selection of adhesives and cleaning agents, as well as influencing long-term decisions about care and storage conditions.
Knowing how far generalisations can be made about the materials used in constructing doped fabric aircraft will, in addition, have major implications for determining how far conservation treatment and collections care decisions can be applied across an entire collection as opposed to on a case-by-case basis.
Beyond the material type, moreover, understanding why aircraft designers and manufacturers selected certain materials provides conservators with a much better insight into the outcomes and results they were hoping to achieve. As noted above, shrinkage of the fabric to make it taut was the primary purpose of applying dope to the fabric, but it was by no means the only consideration. Other requirements of doped fabric are summarised in Table 1, but this list is not exhaustive, and other considerations could be added, such as economy and ease of use.
Table 1
Summary of the requirements of doped fabrics and examples of materials used in their construction
These requirements are important to consider from a conservation perspective since they inform our understanding of how and why an object is significant, both in terms of its tangible and intangible values. They are the intentions and values of the original makers as represented through the materiality of the object itself, and for conservators it is important that there is an awareness of how interventions might affect the way these intentions and values are manifested in the object. A conservation treatment, for example, that sought to stabilise a tear by removing the tautness throughout the doped fabric might be very effective at preventing further tearing, but would have serious ethical implications because of its impact on one of the most fundamental thought processes underpinning the creation of doped fabric aircraft, namely the desire of the original makers to create as taut a material as possible.
The section below discusses in more detail some of the specific materials identified from the historic literature as components of doped fabric, and considers why these were selected by aircraft designers and manufacturers. Furthermore, it considers how and why the presence of these materials may have implications from a conservation perspective.
Fabrics
The selection of fabric was an important consideration for doped-aircraft manufacturers as historic studies found that the properties of the doped fabric were strongly related to the type of fabric yarn selected and its processing to create a finished textile. It seems that there was initially a general preference for Irish linen (Linum usitatissimum), since this was found in experiments to have the greatest tensile strength (Walen, 1918; Esselen, 1918; Turner, 1920). This tensile strength, however, came at the cost of increased stiffness, and some authors argued that cotton (Gossypium hirsutum) would provide a better alternative since, although not as strong as Irish linen under tensile loading, it did have greater elasticity, enabling it to resist tearing under the types of load actually experienced during flight (Walen, 1920; Ramsbottom, 1924).
A third alternative was mercerised cotton. Mercerisation is a process in which the yarn fibres are soaked in strongly alkaline conditions, and recent studies have shown that this causes a reorganisation of the crystalline structure of the cellulose chains that make up the fibre yarns, increasing the amount of hydrogen bonding between polymers (Wertz et al, 2010). Mercerised cotton was, therefore, found to have a greater tensile strength than non-mercerised cotton while still retaining much of its elasticity.
The debate as to which fabric was better appears to have never been truly settled and remained an open question. The USA, in particular, seems to have preferred mercerised cotton over Irish linen, though this may in large part have been down to problems of supply and the greater costs involved in the import of Irish linen to the USA (Catoe, 1962).
The only significant alternative to cotton or linen was silk, although this seems to have been dropped relatively quickly as a material in most countries before the First World War. There may have been a number of factors behind this, such as its high cost and problems of its rapid deterioration in exterior conditions. Another material investigated was ramie (Boehmeria nivea), although this seems to have never received any significant attention or use beyond experimental research (Turner, 1920).
The fabrics discussed above, barring silk, are all derived from naturally occurring plant materials, whose principal component is cellulose. Cellulose is a long-chain polymer molecule in which the separate chains are bonded together by hydrogen bonding (see Figure 4). The properties of cellulose have a major impact on the final characteristics of the different plants that it constitutes. Variations, such as in the length of the cellulose chains, how well ordered and closely packed together these chains are, and whether the cellulose chains are present in a matrix with other materials, mean that different plant materials can have very different material properties (Hon, 1994).
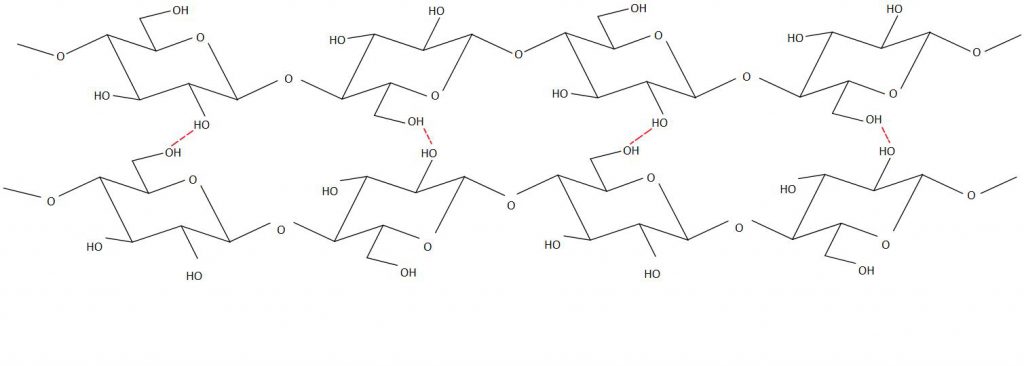
This variation in fabric selection may be of significance to conservation because of the differences in the behaviour and deterioration one might expect from these materials. Cotton, for example, is primarily made of cellulose with relatively few impurities compared with linens, which often contain a much higher proportion of other compounds, such as lignin, hemicelluloses, waxes and oils, depending on how they are prepared and processed (McDougall, 1993).
These impurities have been found to be far less chemically stable than the cellulose content, and increase the likelihood of reactions causing undesired changes in fabrics, particularly under exposure to light radiation and/or oxygen. The main observable alterations might include colour changes, such as yellowing, and a decrease in the tensile strength and flexibility of the material ( Hallett and Bradley, 2003). Of particular concern is the release of acidic compounds by the breakdown of the impurities under exposure to light, since this can lead to acid hydrolysis of the cellulose, which shortens the cellulose chains, leading to a marked reduction in strength ( Seery, 2013).
Exposure to light was observed to be a major cause of deterioration in doped fabrics during the early years of flight, when it was noted that aircraft fabrics deteriorated much faster during summer months compared with winter ones. Further testing using more controlled light sources as well as sending test pieces out to places with high light levels, such as Iraq and Egypt, gradually established that it was the more energetic UV rays of sunlight that were causing most damage (Ramsbottom, 1924; Atkins and Woodcock, 1917; Wendt, 1922; Padfield, 1969).
Besides light, another problem encountered with aircraft fabrics was moisture exposure. During periods of high humidity, moisture uptake would cause fabrics to ‘slacken off’, the tautness being restored once the relative humidity (RH) dropped again and the fabric was able to dry out. This indicates that expansion and contraction cycles of the material occur, which are dependent on cycling of the RH conditions. This type of cycling has been established to be a cause of damage and deterioration in various types of museum materials that conservators deal with, such as ivory, wood and those in paintings, and it seems probable that it would play a part in the deterioration of doped fabric (Michalski, 2011).
Exposure to high RH levels, in addition, risks organic growth developing, such as mould. This is a health and safety issue for those who might have to work with the objects owing to the release of spores into the atmosphere. It can also lead to a weakening of the material, as studies have shown that organic growth on objects can release enzymes that break down the cellulose ( Edwards and Falk, 1997). Such a process causes the long-chain molecules of the cellulose to break down, reducing the structural integrity of the material.
Dope polymers
The selection of the polymer to be used in dope formulations was very important as it was this polymer that would form a film within and around the fabric fibres, causing it to shrink and grow taut. Early, pre-doped fabric aviators experimented with a number of readily available options, such as casein, glues and resins, but none were found to be particularly effective (Drinker, 1921; Smith, 1919).
The main group of materials discovered to be effective tauteners was cellulose esters. These are semi-synthetic plastics, in which the cellulose chain is modified by replacing a proportion of the hydroxyl groups attached to the main chain with a new chemical group (see Figure 5). Depending on the type of group used to replace the hydroxyl group, a very large number of products is theoretically possible, whose properties may further vary depending on factors such as how many hydroxyl groups are replaced, the distribution of the substituent groups along the chain, the length of the polymer molecules and the presence of impurities from the production mechanism (Zugenmaier, 2004).

Cellulose nitrate was the first cellulose ester to be used in aircraft dopes in the years before the First World War. It was a major step forward, having very good tautening powers, and set the standard against which alternative polymers would be judged for years to come (Kline and Malmberg, 1938; Reinhart and Kline, 1939; Inoue, 1941). It was, however, also highly flammable, and so more stable alternatives were sought, which led to the development of dopes based on cellulose acetate, cellulose acetate butyrate and cellulose stearate.
Each of these cellulose derivatives has different properties and characteristics that provided them with various advantages and disadvantages. No definitive decision was ever made as to which polymer was best for tautening and all – except cellulose stearate, which appeared too late in the history of doped fabric to be widely investigated or adopted – were used in many different contexts and parts of the world (Weissberg and Kline, 1949; Meyer and Gearhart, 1944; Dreyfus, 1949; Jones and Hockney, 1944).
The presence of these cellulose-derived materials is a potential challenge from a conservation perspective. All of these polymers are potentially unstable, undergoing chemical degradation on exposure to various conditions, such as UV light, heat and moisture. The result of such chemical changes might be the loss of substituent side groups from the polymer chain or even scission of the main polymer chain itself, leading to a drop in molecular weight (Berthumeyrie et al, 2014; Quye et al, 2011; Selwitz, 1988). These reactions can have a significant effect on various properties of the material, such as its colour, tensile strength, flexibility and acidity.
As with cellulosic fabrics, light is particularly damaging to objects containing cellulose derivatives. This is because the UV energy is sufficient to break the bonds holding the side groups – the acetate, nitrate or butyrate groups – to the cellulose polymer backbone. Once released, these chemical groups are then able to react with moisture to form acidic compounds. These acidic compounds then react with the main polymer cellulose chain, causing it to break down and shorten.
This has serious consequences for the properties of the material, since it affects the length of the polymer chain molecules. As these shorten, the tensile strength of the material is decreased as there is a greater number of weak points in the material where failure might occur. It can also result in a less extensible and more brittle material, further increasing the risk of failure. This is because the areas most vulnerable to degradation reactions tend to be amorphous portions of the material from where much of the material’s flexibility is derived, and the reactions may also lead to cross-linking of the polymer chains, which again limits their ability to move past and around each other ( Hon and Gui, 1986).
This history of the material itself, in terms of how it was produced and its quality, is also highly relevant to the issue of its conservation. When making cellulose derivative products, sulphuric acid is often used as a catalyst in order to substitute the hydroxyl groups of the cellulose chain with the required chemical group. If the final product is not then properly purified, trace sulphuric acid can remain in the material, again leading to acid hydrolysis and chain scission of the cellulose backbone.
Dope additives
Besides the polymer of the dope responsible for forming a film, numerous other compounds might be added to manipulate the film in various ways. These could include plasticisers, fire retardants, pigments, UV reflectors and absorbers, and fungicides (see Table 1). The list of materials that might be added therefore includes, but is not limited to, items such as triphenyl phosphate, castor oil, aluminium powder, iron oxide powder, a boric acid and borax mixture, and inorganic pigments such as carbon black and yellow ochre. The proportions of the mixtures would also vary, some having higher quantities of the different components, depending on the particular manufacturer and dope purpose.
Use of these additives significantly complicates the potential long-term behaviour and predictability of the material, as it introduces further potential reactions and processes. Metallic compounds, for example, have been found to act as catalysts in the degradation of other cellulosic materials, and triphenyl phosphate degradation is believed to potentially release phosphoric compounds which may form acids, again causing and increasing the rate of degradation reactions (Daniels, 1999; Tsang et al, 2009; Groom, 1999).
As well as the dope ingredients, the solvent mixture in which it was all dissolved in order to be brushed or sprayed onto the fabric could also vary significantly. Drinker (1921) reports that over fifty solvent formulations were in use at various times by the Allies during the First World War alone, which could result in unintended and unanticipated consequences. Tetrachloroethane, for example, was a common solvent in use in most countries prior to 1916, but research at the Royal Aircraft Factory indicated that when trapped within the material it could react in sunlight, releasing acidic volatile compounds which then reacted with the fabric substrate, increasing its rate of deterioration (Robertson, 1916; RAF, 1915). This, coupled with the fact it was linked with several fatalities and numerous health issues, meant it was phased out of British dopes in 1917 (Hamilton, 1918).
Other products, such as varnishes and paints, might also be combined with the doped fabric to act as a protective coating against environmental conditions. These varnishes created a waterproof finish, and could be pigmented with metal powders or other compounds to absorb and reflect sunlight before it could reach the doped fabric. There were numerous varnishes developed during the First World War, such as Protective Coating 10 (PC10), which was a nitrocellulose-based varnish containing carbon black and yellow ochre pigments, and V84, which was a nitrocellulose varnish containing aluminium powder to absorb and reflect the energy from the Sun’s rays (British Engineering Standards Association, 1918).
The documents stored at the NA make clear, however, that this was an area of constant change and development as new ideas and supply issues meant that new materials had to be developed. In 1918, for example, two new products named pigmented oil varnish (POV) and clear oil varnish (COV) were introduced which were made with tung oil (Vernicia fordii) and intended to replace varnishes such as PC10 and V84 because of a shortage of nitrocellulose.[1]
Towards the end of the First World War, the British also began experimenting with pigmenting dopes directly by adding aluminium powder straight into the dope formulation. This obviated the need to add any varnish or protective coat over the doped fabric, and in time appears to have become the most widely accepted method for providing environmental protection. Such pigmented dopes, however, would only usually be applied in the very top two coats to save on weight, with the first few coats below remaining unpigmented.
The dope, therefore, not only made the fabric taut, but protected it from environmental factors that might cause it to weaken and fail. In this role it acted as a sacrificial material designed to protect the more expensive fabric below, and was expected to deteriorate in the environment. A time frame of 60–90 days was suggested before the dope should begin to show any negative signs of ageing, such as peeling away from the fabric and discolouration, when it would require replacing (Esselen, 1918). This raises an interesting conservation question as to whether conservators should necessarily attempt to preserve dope for as long as possible, or should be willing to renew it periodically given the transient nature of the material.
Doped fabric summary
From the above discussion it appears that some general assumptions can be made about doped fabrics, primarily that they will consist of some type of cellulosic fabric impregnated with a cellulose ester-based compound. It is also important to be aware of some recurring causes of deterioration. Exposure to light in particular can be highly damaging to doped fabric materials, as can exposure to fluctuating RH. More research, however, is required to establish the deterioration mechanisms taking place and how these alter the characteristics of doped fabrics.
On the other hand, these generalities still mask a very high degree of variability, with different materials and processes used in different times and locations. A few examples of dope recipes proposed and used in various times and parts of the world are provided in Table 2. These examples help to indicate the range of materials used just within the dope, but it is also worth remembering that this variability extends to other decisions such as the choice of fabric and the application of protective varnishes.
From a conservation perspective, this means that caution is required when devising and applying treatment methodologies. Differences between doped fabrics may mean that a treatment that is effective in one context will not work as well in another. It may also mean that types of deterioration that occur for one object will not necessarily be common or expected across all types of doped fabric aircraft.
Table 2
Examples of different dope formulations*
Current conservation of doped fabric
https://dx.doi.org/10.15180/160605/012The specific context in which a conservation decision is made is very important since it is what will ultimately determine the final outcome of the treatment. Factors such as the particular history of the object, the prevailing philosophies at a particular time, the reason an object is receiving conservation, the ethos and aims of the owners, the training and skills of those involved and resources available will vary between projects and influence the approach taken. It is therefore impossible to provide a definitive ‘correct’ approach. The following discussion, instead, presents several options available to manage the conservation of doped fabrics, and an evaluation of their potential strengths and weaknesses from a museum conservation perspective.
From the examples that are available within the literature and examples of ongoing projects, it seems that re-covering of aircraft was historically the most common approach within museum collections and remains a viable option now (Mikesh, 1997; Firth, 2009).[2] This practice, whereby the old material is removed and replaced with new, ensures that objects have a high aesthetic appeal in that they do not show signs of wear and tear or damage, and are ready for public display.
Re-covering also provides an opportunity to examine the interior surfaces and parts of an aeroplane, where other forms of deterioration may have taken place unobserved beneath the doped fabric covering. The removed material, moreover, may be easier to handle, pack into a smaller space and store in more controlled conditions, compared with when it must be maintained in situ on the object, and so there is the possibility that the material can be preserved for much longer out of context, and made more easily available for research projects and study.
The process of re-covering, however, raises a thorny issue in conservation discussions as to the distinction between a restoration and a conservation approach, and whether such a major intervention necessarily fits as a conservation treatment. The distinction between conservation and restoration can be subtle and hard to clarify, but probably comes down to a matter of approach and how the aircraft is re-created and reinterpreted before, during and after the treatment process ( Staelens and Morris, 2010; McManus, 1994; Horelick, 2015; Croker and Kemister, 2009).
In a conservation treatment, one of the most important aspects is not necessarily the actual physical work done to the object, but the work that goes into understanding the object in terms of what are often described as its tangible and intangible values, or its significance (Australia ICOMOS, 1999; Wharton et al, 2011). This relates to understanding how and why an object is important in terms of both the physical materials from which it is made, and the more ephemeral cultural or social ideas that may be associated with it. It is only through understanding these ideas that an informed conservation plan can be agreed, in which it is recognised that conservation will at once enhance, but also potentially destroy, certain values associated with an object (Odegaard, 1995).
Removing signs of damage from an object, for example, may restore a previous aesthetic appearance, but simultaneously it removes a part of that object’s history, namely evidence of the fact that it was damaged. It is only through understanding how and why the damage occurred, and how this relates to other values held about the object, that its contribution to our interpretation of the object can be properly understood. A conservation treatment, therefore, may well resolve that retaining signs of damage is in itself a good thing.
A restoration treatment, in contrast, will potentially start from a very different conceptual understanding of the object, in that it will seek to return it to a perceived earlier state, and this concept of the object’s earlier form will take primacy over all other considerations. There will not, therefore, be the same attention paid to trying to understand the object as fully as possible and explore alternative treatment options beyond this one interpretation. The key difference from conservation, therefore, is not that the actual treatment will necessarily be different, but rather lies in the paths and decision-making processes used to reach that final treatment decision (Vinas, 2005, p 27).
A re-covering, therefore, needs to be very carefully thought through and justified if it is to be an effective conservation treatment, as opposed to a restoration, and there are a number of dangers that need to be considered. The desire to source historically appropriate materials, and carefully re-create original stitch patterns for example, may, counter-intuitively, be problematic since the production of aeroplanes was not always of the highest quality or standard owing to variability in production and manufacturing.
Painstakingly re-covering an aircraft, therefore, attempting to get every single detail perfectly correct and looking pristine, risks losing the very idiosyncrasies and imperfections that perhaps made the object unique and gave it its identity. The end result, therefore, is an idealised object that has, for want of another word, been made ‘better’ than it ever was in previous incarnations. This would be classed more as a restoration and not a conservation, since the focus is on returning the aircraft to an earlier form, even though in this case it never actually existed, without adequately thinking through what this might mean for a viewer’s interpretation and understanding of that particular object.
There are, in addition, a number of practical considerations that may limit the desirability of re-covering in certain contexts. Practically, it requires a large enough space in which to work, and a doping area fitted with special ventilation and environmental control systems for health and safety purposes, as well as to control the dope drying process. Sourcing materials representative of those being replaced can be a further difficulty, and finding staff with the required skills and knowledge can also be challenging. The process of re-doping, moreover, presents potential danger to the underlying frame. Excessive shrinkage can occur in the doped fabric which may distort other parts of the object, and there is anecdotal evidence that cellulose acetate butyrate dopes in particular can continue shrinking long after the initial application (Pearce, n.d.).
An alternative, less interventive option for dealing with tears in the fabric of aeroplanes is to cover the tear with a patch of material, and then secure this in place with several coats of fresh dope.
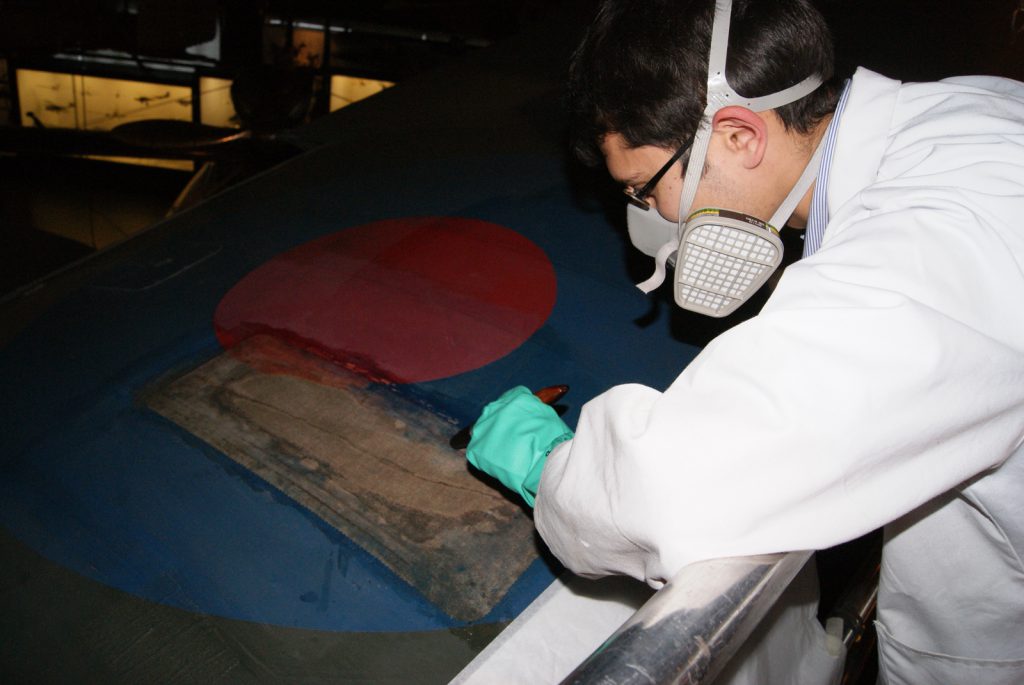
Depending on the quality of the treatment, this approach appears to result in a strong bond between the patch and the area around the torn doped fabric surface, and so prevents further growth of the tear. The tautening effect of the dope used in the repair also maintains the profile of the wing as it restores the stretched, smooth profile, which may be lost owing to the release of tension when the tear occurs. It is, finally, a simple, easy method that requires relatively few resources, and can result in an aesthetically unobtrusive result as the new materials match the original but can still be distinguished from the original on inspection. It also maintains traditional skills and knowledge regarding the maintenance of historic aircraft.
The use of doped patches, however, can also cause further problems. The shrinking of the doped patch introduces more strain into the material, which may simply cause the doped fabric to begin tearing in a new area – a result which is unacceptable for a conservation treatment. The use of patches, moreover, can become very aesthetically distracting if there are many areas requiring treatment, and a surface may end up resembling a patchwork quilt rather than a smooth flying surface.
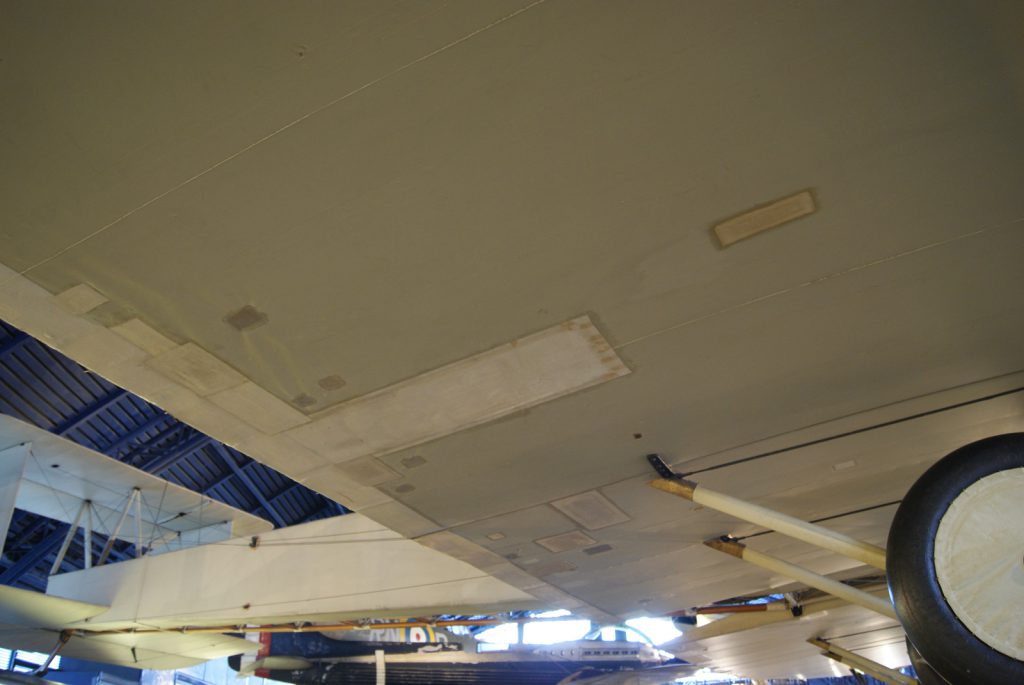
Applying and removing a doped-patch area, moreover, without significantly affecting the underlying doped fabric surface of the aeroplane, is almost certainly impossible as the solvents used in the fresh dope will probably have an effect on the underlying surfaces of the museum object. This is very important to conservation treatments since the idea of reversibility or re-treatability, whereby any conservation work should be removable from the object without significantly altering the object that is left behind, is a core concept that helps inform many conservation decisions (Lambrinou, 2010).
In order to further inform this aspect of the treatment, however, much better knowledge is needed about how different conservation materials might react and behave when left in long-term contact with doped fabric. The ideal would be to find a material that could be applied in a form that would not chemically interact with the underlying substrate, and would still be removable at a future date without causing significant change to the original doped fabric material.
Aside from the idea of reversibility, another key concept for conservators is that of minimum intervention. This idea states that conservators should only do as much intervention as is required for the desired outcomes. In this particular instance it has already been discussed how the patches are effective at achieving the required outcome of stabilising the tear, but there is a question whether they are still too intrusive. It is not clear, for example, that it is necessary to cover the entire area around a tear with a patch or if alternative, smaller repairs might prove just as effective at preventing tear growth. Understanding of the forces acting on the tears, therefore, and the strength, position and size of any repairs necessary to effectively stabilise these forces would be required to adequately inform this part of any new treatment proposal.
Caution must also be exercised in using modern commercial dopes, since they are proprietary formulations and not all the ingredients are known. This is obviously a problem in using any proprietary conservation material, but is especially true at present for modern commercial dopes as there has been so little study of their short- or long-term behaviour as conservation materials. Their compatibility for use with other dopes, moreover, should not be taken for granted.[3]
Alternative patching materials have been investigated at the Science Museum in a student project undertaken on the Vickers Vimy (Beesley, 2009). This sought to test the efficacy of materials already well known within the conservation profession, such as Beva 371 (unknown proprietary formulation) and Lascaux 498HV and 360HV (both acrylic polymers based on butyl methacrylate). Unfortunately these repairs are showing signs of failure where they have been put into practice, indicating that either the materials or techniques used to apply them lack sufficient long-term performance in this context.
Doped fabric aircraft at the Science Museum
https://dx.doi.org/10.15180/160605/013As discussed above, for conservation to be successful it requires a thorough understanding and consideration of an object’s value and significance. This requires knowledge of the object’s history, how it may have been interpreted and used at different times, and an appreciation of its current setting, condition and context. This section therefore provides an overview of the aircraft in the Science Museum’s collection, assessing how they are currently displayed and the archival materials of relevance kept in the Museum.
The majority of information relevant to the Museum’s aircraft is kept in technical files, where notes, articles and correspondence about the objects are maintained and added to, usually by curatorial staff. Information of relevance to this project kept in the technical files for three of the Science Museum’s doped fabric aircraft is summarised in Table 3 (see Appendix for details of additional doped fabric aircraft).
Table 3
Summary of information found in the Science Museum’s technical files relating to three of the Museum’s doped fabric aeroplanes
The majority of the Museum’s doped fabric aeroplanes are on display in the main museum at South Kensington in the Flight gallery. This gallery has little in the way of environmental control and an initial analysis of environmental data indicates considerable fluctuations in RH over time which, as discussed above, could be contributing to deterioration. Many of the aircraft are suspended from the ceiling out of reach of visitors, yet several, notably the Vickers Vimy and Supermarine S6B, are at floor level and easily within reach.
The majority of aircraft not on display at South Kensington are in storage at the Science Museum’s large-object storage facility at Wroughton, Swindon. This is a former airfield, and the aircraft are kept in repurposed aircraft hangars. These provide varying levels of protection in the way of environmental control for the aircraft, and more details of the site, and the environmental monitoring and research being undertaken there, have recently been published (Leskard, 2015). This environmental monitoring is important so that conservators can better understand the conditions in which the objects are kept and, using knowledge of how the environment can induce degradation reactions, make decisions on how the environment might be altered to limit them.
There does not appear to have been an explicit strategy or objective in the collection of aircraft at the Science Museum. Aircraft have been collected and considered significant for a variety of different reasons. Several are valued because of their role in specific events in aviation history or for their connection to a particular individual. Such aircraft include the Vickers Vimy, which made the first transatlantic crossing in 1918, and the Supermarine S6B, which won the Schneider Trophy in 1931.
Other aircraft within the collection are significant because they provide information about ongoing and broader processes in aviation practice. This might include both technological development, such as early flight designs, and changes in social practice, such as early aircraft for mass transport and commercial travel. The collection therefore has no single interpretation but represents numerous different aspects of flight, in terms of its social history and technological development.
It is difficult to assess the extent of previous conservation, restoration and repair work carried out on the Science Museum’s aircraft owing to a lack of detailed record-keeping up until the 1990s. It is important to gather as much information as possible about these earlier interventions, however, in order to make judgments about concepts such as the ‘originality’ or ‘authenticity’ of the objects. This is important as it could have a meaningful impact on how the values of the objects are interpreted – for example how important it might be in any given treatment to ensure the doped fabric is left unaltered and intact, or whether it could be treated more drastically if not felt to be ‘authentic’ or of great significance.
Correspondence from the technical files makes clear that many of the aircraft donated in the 1920s and 1930s were re-covered when accessioned into the Museum collection. This might have been for several reasons, such as repairing damage from the working life of the object or simply to improve its appearance. Unfortunately specific details of these re-coverings, such as the materials or processes used, and how closely these were meant to match the earlier doped fabric, are rarely given.
The technical files indicate that the majority of doped aircraft at the Museum were then again re-covered in the 1960s and 1970s to prepare them for display in the new Flight gallery. Some of this work seems to have been undertaken by apprentices at the original manufacturing companies who built the aeroplanes, such as at Hawker.[4] Again, however, no documentation was kept regarding the materials used or the extent to which original techniques were used in these re-covering practices.
In addition to these re-coverings, ad hoc interventions have been undertaken by Museum staff over time. It seems that the aircraft were treated by re-doping them periodically to re-tauten and stretch the fabric. A note from an external adviser researching the colours of the Hawker Hurricane in the 1970s makes clear that, in his view, staff planned to apply inappropriately pigmented dopes to re-tauten the fabric of aircraft on display.[5] It is not stated which, if any, of the dopes the Museum staff actually applied or how often this process might have been undertaken.
It is also likely that doped patches were attached to cover tears, given the aged appearance of some of these interventions, though no references to this have been found in the documentation, and it is not clear who applied them or when. In more recent times the documentation and recording of intervention work carried out on aircraft has improved significantly, and the current approach is still to use doped fabric patching, as discussed above.
The interpretation of the Science Museum planes, therefore, and the elucidation of their value and significance, is a complex process. The objects certainly represent a very important, varied and significant collection, but their treatment and management means that the fabric coatings on most aircraft are almost certainly not original in the sense of having been in use when the aircraft were operational or first produced; though exactly how much intervention has taken place over the years is generally unclear.
This raises interesting ethical questions regarding the doped fabric material on the aircraft, such as how far it should be valued for its own sake, and how justifiably it might be removed, patched or left untreated without fundamentally changing the interpretation or significance of the objects. As stated above, such debates often have no objectively correct answer, but require thought and discussion if an informed conservation decision is to be achieved.
Conclusions
https://dx.doi.org/10.15180/160605/014The Science Museum collection of doped fabric aeroplanes is clearly of significance and interest. Unfortunately, however, records pertaining to its early history and conservation management are sporadic and were poorly kept, making it very difficult to securely construct a coherent conservation history for all of the objects. Much of this early information is based on passing reference and suggestions contained within correspondence between Museum staff, rather than explicit reports, so it is unclear exactly how many times or with what materials the objects may have been re-covered or re-treated over the years.
The complexity of reconstructing what types of materials may be present on the Science Museum aeroplanes is further complicated as doped fabrics represent a complex set of materials, whose composition varies widely depending on a number of factors. There is no single definitive version of doped fabric, which is in fact a broad family of related materials, which makes conservation work much more complex. A treatment approach that may be appropriate in one context with one particular doped fabric could in theory react differently when used on another doped fabric because of the differences in material nature and history.
It is clear, however, that there are a number of common materials that might generally be expected to be present in a doped fabric. This will make the process of scientific analysis considerably more straightforward, as it provides a starting point from which to begin investigative work and hypotheses concerning the material. This will form the next stage of the project, characterising and testing the properties of the doped fabric.
Acknowledgments
The authors are grateful to the Arts and Humanities Research Council for funding this research project through the Collaborative Doctoral Partnership scheme. Thanks are also owed to the staff in the Science Museum’s conservation and documentation departments for enabling access to the archives and aircraft. Our thanks also to Darren Priday and the conservation team at the Royal Air Force Museum, Cosford, for taking the time to discuss and demonstrate their work treating historic aircraft.
Appendix
https://dx.doi.org/10.15180/160605/015Table of doped fabric aircraft studied using the Science Museum’s technical files
Tags
Footnotes
Back to text
Back to text
Back to text
Back to text
Back to text